研究背景
外来物种入侵是当今全球化时代导致生物多样性下降的重要因素(Simberloff et al., 2013; 魏辅文等, 2014),世界自然保护联盟(IUCN)公布的782种已绝灭的动物物种里261种(33%)与外来物种入侵紧密相关(Blackburn et al., 2019)。同时,外来入侵物种还给当地经济带来重大灾难,最新数据显示,过去几十年(1970-2017年),全球报告的外来入侵物种导致的经济损失至少有1.288万亿美元,年平均损失为268亿美元(Diagne et al., 2021)。我国是受生物入侵危害最严重的国家之一(丁建清, 2008; 万方浩等, 2009; 李博和马克平, 2010; Bailey et al., 2020),生态环境部门公布的数据显示,目前我国已记录到的外来入侵物种高达600多种,科学管控外来物种入侵已成为国家重大发展战略。纵观“引种-建群-扩散”三个主要生物入侵阶段(Blackburn et al., 2011),由于外来物种一旦建立野生繁殖种群便很难完全根除(Simberloff et al., 2013),因此,如何预测并防控外来物种的早期入侵风险对制定经济有效的生态安全对策十分重要(Fournier et al., 2019)。其中,由于物种的基础生态位(Fundamental niche)通常难以量化,以物种占据的实际生态位(Realized niche)如气候实际生态位(以下简称气候生态位)为依据的物种分布模型(Species Distribution Modeling,SDM)是当前外来物种入侵风险的主要预测工具(Guisan et al., 2013),其理论基础是气候生态位保守性假说(Peterson, 2011),即物种气候生态位在空间和时间上趋于保守(不变)的特性。然而,物种生态位保守性假说一直存在很大争议(Losos, 2008; Wiens et al., 2010),以外来物种的气候生态位为例,基于北美入侵到欧洲和澳洲的外来植物(Petitpierre et al., 2012)、欧洲外来鸟类(Strubbe et al., 2013)等的研究发现,这些类群在入侵过程中的气候生态位趋于保守,但越来越多的证据如外来两栖爬行动物(Li et al., 2014; Liu et al., 2017, Tingley et al., 2014),一些全球性外来植物(Atwater et al., 2018)、入侵到地中海的印-太平洋热带鱼类(Parravicini et al., 2015)、一些全球性外来鸟类(Cardador and Blackburn, 2020)在入侵过程中都会发生气候生态位的快速变化(即漂移,niche shift)。造成这一争论的重要因素之一是外来物种气候生态位的量化方法(如背景空间的选择、空间分辨率偏差和采样误差等)在不同研究中的差异及长期缺少一个统一的量化框架(Fitzpatrick et al., 2008; Peterson and Nakazawa, 2008; Bates and Bertelsmeier, 2021; Liu et al., 2021)。因此,了解和掌握外来物种在入侵过程中气候生态位动态的量化方法,对有效解决生态位保守性之争、发展更加准确的外来物种早期入侵风险预警框架、评估外来物种和本土物种面对未来气候变化下的分布区动态都极为关键。
外来物种在入侵区的气候生态位动态量化方法经历了多个发展阶段,主要包括早期的简单基于物种地理分布空间的气候平均值比较(Rödder and Lötters, 2009)到近年来基于物种环境空间的气候密度比较的方法(Broennimann et al., 2012)。具体来看,瑞士洛桑大学Antonie Guisan教授团队为了控制物种分布采样偏差对结果的影响,首次将物种的地理空间转化为环境(气候)空间并将其网格化,进而将核平滑函数应用于物种环境空间并计算物种占据气候生态位的空间密度,利用经典Schoener D指数(Schoener, 1970)度量了原产地和入侵地范围内物种气候密度的重叠暨相似程度(Broennimann et al., 2012)。在此基础上,该团队继续以外来植物为例,创造性的将外来物种在入侵区和原产地的真实气候生态位动态的关系量化为缺失(Unfilling)、稳定(Stability)和扩张(Expansion)三部分,并严格定义和论证了只有扩张(Expansion)部分才为真实气候生态位的漂移(Petitpierre et al., 2012; Guisan et al., 2012)。这一方法历史性的改变了学界对外来物种气候生态位入侵区和原产地存在差异的传统认知,准确捕获到了气候生态位真正发生变化的部分(Guisan et al., 2014)。该量化框架发表以后,受到了学界极大反响并被广泛应用于各种外来生物类群在入侵区的气候生态位动态研究之中,目前谷歌学术搜索已被引用660余次。本文以一种全球性外来入侵两栖类——美洲牛蛙(Lithobates catesbeianus = Rana catesbeiana)为例,详细介绍外来物种在入侵区气候生态位动态的量化方法和计算流程。
软件版本信息和下载地址
- R software version 4.0.4 (https://www.r-project.org)
- rgeos version 0.5-8 (https://CRAN.R-project.org/package=rgeos)
- raster version 0.5.8 (https://CRAN.R-project.org/package=rasterList)
- ecospat version 3.2 (https://CRAN.R-project.org/package=ecospat)
- maps version 2.3.0 (https://CRAN.R-project.org/package=mapdata)
分析流程
本案例分析包括如下过程:物种分布和气候数据收集、二维网格物种地理空间和环境空间的定义、应用核平滑计算二维环境空间的气候密度、通过随机检验方法对原产地和入侵区气候生态位的相似性进行统计检验,量化入侵区相比原产地的气候生态位动态(图1)。
图1. 外来物种气候生态位动态量化流程框架图
一、物种分布数据和环境图层数据收集
美洲牛蛙的分布点数据来自各种数据库和文献(Liu et al., 2020),共计收集原产地分布点信息1337条和入侵区分布点信息109条。根据Kraus(2009)的标准划分原产地和入侵区范围,并根据Olson et al.(2001)的生物地理区系划分标准来定义物种气候生态位动态量化分析的背景空间(Li et al., 2014),该物种原产地所在生物地理区系为新北界(Nearctic),本文使用的入侵区生物地理区系为新热带界(Neotropical)。气候图层来自WorldClim(https://worldclim.org/data/worldclim21.html),分辨率设为50 km × 50 km,选取温度和降水变量(具体见下文,Araújo et al., 2008; Li et al., 2014)。
二、在R软件中量化气候生态位动态
- 读取分布点数据
打开R软件,安装以下函数包:rgeos,raster,ecospat,maps。
install.packages("rgeos")#安装rgeos函数包,安装raster,ecospat,maps函数包,可输入相同的命令
在进行数据分析前,指定数据存放的工作目录,然后在R软件中设置工作目录并读取数据。
读取分布点数据
mypath0 <- "" ## 设置工作目录
locs.native <- read.delim(file = paste0(mypath0, "/data/bull_na.txt"))#读取原产地分布点信息
locs.invasive <- read.delim(file = paste0(mypath0, "/data/bull_nt.txt"))#读取入侵区分布点信息
colnames(locs.native) <- colnames(locs.invasive) <- c("lon", "lat") #修改数据名称
maps::map('world', col = 'grey85', fill = T, border = 'grey85')
points(locs.native, pch = 20, col = "darkgreen")#深绿色圆点表示原产地分布点
points(locs.invasive, pch = 20, col = "red")#红色圆点表示入侵区分布点(图2)
图2. 用于本文的外来入侵物种—美洲牛蛙的原产地和入侵区分布点数据
- 读取气候图层数据
指定数据存放的工作目录,本案例选择了前期研究中使用的对两栖动物分布和生理有重要影响的8个气候变量(Liu et al., 2019, 2020):包括年平均温度(bio1)、气温季节性变化(bio4)、最热月份的最高气温(bio5)、最冷月份的最低气温(bio6)、年降雨量(bio12)、降水季节性变化(bio15)、最湿润季度的降雨量(bio16)和最干燥季度的降雨量(bio17)。背景空间定义使用最小凸多边形(Minimum Convex Polygon, MCP),并以美洲牛蛙的主要自然扩散距离(Austin et al., 2003; Wang et al., 2022)为依据在其周围设置一个2弧度的缓冲区。
env.files <- list.files(path = paste0(mypath0, "/data/wc2.1_10m_bio"), pattern = 'tif',full.names = TRUE)
env.rasters <- stack(env.files)
final.var <-c("bio1", "bio4", "bio5", "bio6", "bio12", "bio15", "bio16", "bio17")#选取与温度和降雨相关的8个气候变量:年平均温度(bio1),气温季节性变化(bio4),最热月份的最高气温(bio5),最冷月份的最低气温(bio6),年降雨量(bio12),降水季节性变化(bio15),最湿润季度的降雨量(bio16),最干燥季度的降雨量(bio17)。
rasters.selected <- stack(subset(env.rasters, final.var))
mcp <- function (xy) {xy <- as.data.frame(coordinates(xy))coords.t <- chull(xy[, 1], xy[, 2])xy.bord <- xy[coords.t,]xy.bord <- rbind(xy.bord[nrow(xy.bord), ], xy.bord) return(SpatialPolygons(list(Polygons(list(Polygon(as.matrix(xy.bord))), 1))))}
buffer.size <- 2#最小凸多边形(MCP)周围设置2度的缓冲区 - 主成分分析(Principal Component Analysis)
将物种原产地和入侵区的气候因子合并进行主成分分析(Broennimann et al., 2012; Li et al., 2014)。
locs.env<-cbind(rbind(locs.env.native,locs.env.invasive),species = c(rep("native", nrow(locs.env.native)), rep("invasive", nrow(locs.env.invasive))))#分布信息
back.env<-cbind(rbind(back.env.native,back.env.invasive),species=c(rep("native", nrow(back.env.native)), rep("invasive", nrow(back.env.invasive))))#背景信息
data.env <- rbind(locs.env, back.env)#环境值的合并数据
pca.w <- c(rep(0, nrow(locs.env)), rep(1, nrow(back.env)))#权重矩阵
pca.env <- dudi.pca(data.env[, final.var], row.w = pca.w, center = TRUE, scale = TRUE, scannf = FALSE, nf = 2)
ecospat.plot.contrib(contrib = pca.env$co, eigen=pca.env$eig)#使用ecospat函数包绘制各个变量的贡献值,线条长度表示贡献值的大小,如图3。
图3. 各个变量的贡献值。
- 物种原产地和入侵区气候生态位网格化
根据观测到的物种分布密度和气候背景空间,分别创建基于物种分布密度的原产地和入侵区的气候密度网格,这部分操作将有助于控制物种分布的采样偏差对结果的影响。
网格化原产地生态位
grid.clim.native <- ecospat.grid.clim.dyn(
glob = pca.scores.back.glob, ##整个研究区域的背景
glob1 = pca.scores.back.native, ##物种分布的背景空间像素
sp = pca.scores.native) ##物种的环境值
网格化入侵区生态位
grid.clim.invasive <- ecospat.grid.clim.dyn(
glob = pca.scores.back.glob, ##整个研究区域的背景
glob1 = pca.scores.back.invasive, ##物种分布的背景空间像素
sp = pca.scores.invasive) ##物种的环境值 - 生态位重叠
使用相似度检验来计算D metric。D值在0-1的范围,0代表原产地和入侵区的生态位完全没有重叠,1则代表完全重叠。
D.overlap<-ecospat.niche.overlap(grid.clim.native,grid.clim.invasive,cor=TRUE)
D.overlap$D; D.overlap$I
除了要量化外来物种气候生态位在原产地和入侵区的重叠程度,一般还需要进行生态位一致性检验和相似性检验(Broennimann et al., 2012)。一致性检验一般测试原产地和入侵区的气候生态位是否等同。
eq.test <- ecospat.niche.equivalency.test(z1 = grid.clim.native, z2 = grid.clim.invasive, rep = 10, alternative = "greater", ncores = 3)
生态位相似性检验则主要测试物种的原产地和入侵区的生态位是否比预期的更相似,即原产地生态位与入侵区生态位的重叠程度是否高于随机引入时的重叠程度。
sim.test <- ecospat.niche.similarity.test(z1 = grid.clim.native, z2 = grid.clim.invasive, rep = 100, alternative = "greater",rand.type = 1)
由分析可得生态位重叠指数D = 0.1338,相似性结果为0.1832,均小于0.2,说明原产地和入侵区的生态位重叠十分有限(Rödder and Engler, 2011)。 - 生态位动态量化
量化气候生态位动态三部分:扩张(漂移),稳定和缺失。
niche_COUE <- ecospat.niche.dyn.index(z1 = grid.clim.native, z2 = grid.clim.invasive, intersection = NA)
niche_COUE$dynamic.index.w得到扩张(漂移)为0.6056、稳定为0.3943,缺失为0.8685。
par(title = "Niche Overlap", name.axis1 = "PC1", name.axis2 = "PC2")
ecospat.shift.centroids(sp1 = pca.scores.native, sp2 = pca.scores.invasive,col = "black")得到如下图示结果(图4),蓝色部分表示稳定、绿色部分缺失、红色部分表示扩张(即漂移)。
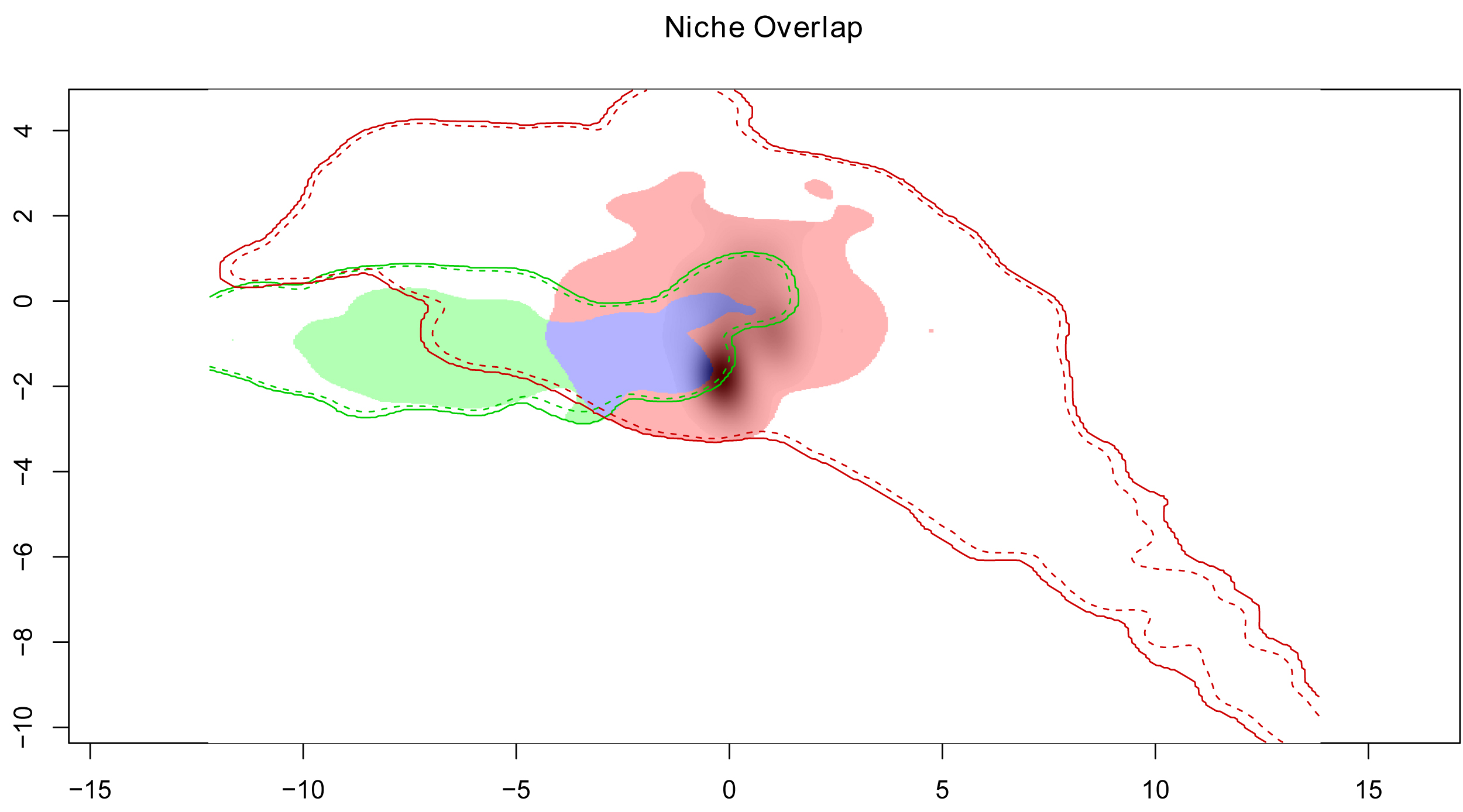
图4. 入侵种美洲牛蛙从新北界原产地到新热带界入侵区的气候生态位动态图
因此,我们可以得出结论,样例物种(美洲牛蛙)在原产地范围和入侵地范围之间没有显著的气候生态位保守性。
本案例以美洲牛蛙为例,介绍了外来物种在入侵区的气候生态位动态量化方法,读者可通过R软件结合物种大尺度分布数据和气候数据,量化外来物种在入侵过程中的实际气候生态位动态变化,支撑基于物种分布模型的外来物种潜在入侵风险预测。本文以入侵两栖类为例,相关方法量化框架可以在不同时空尺度和不同生物类群中被广泛应用。
致谢
本项工作得到国家自然科学基金(32171657,31870507),第三次新疆综合科学考察项目(2021xjkk0606),中国科学院青年创新促进会(Y201920)和南疆经济与社会高质量发展研究项目(NFS2101)的支持。
竞争性利益声明
作者声明没有利益冲突。
参考文献
- 丁建清. (2008). 应对外来生物入侵挑战,促进可持续发展. 中国科学院院刊23: 330-335.
- 李博,马克平. (2010). 中国学者面临的转化生态学机遇与挑战. 生物多样性18: 529-532.
- 万方浩,郭建英,张峰. (2009). 中国生物入侵研究. 科学出版社,北京.
- 魏辅文,聂永刚,苗海霞,路浩,胡义波. (2014). 生物多样性丧失机制研究进展. 科学通报59: 430-437.
- Araújo, M. B., Nogués-Bravo, D., Diniz-Filho, J. A. F., Haywood, A. M., Valdes, P. J. and Rahbek, C. (2008). Quaternary climate changes explain diversity among reptiles and amphibians. Ecography 31: 8-15.
- Atwater, D. Z., Ervine, C. and Barney, J. N. (2018). Climatic niche shifts are common in introduced plants. Nat Ecol Evol 2: 34-43.
- Austin, J. D., Davila, J. A., Lougheed, S. C., and Boag, P. T. (2003). Genetic evidence for female-biased dispersal in the bullfrog, Rana catesbeiana (Ranidae). Mol Ecol 12: 3165-3172.
- Bailey, S. A., Brown, L., Campbell, M. L., Canning-Clode, J., Carlton, J. T., Castro, N., Chainho, P., Chan, F. T., Creed, J. C., Curd, A. et al. (2020). Trends in the detection of aquatic non-indigenous species across global marine, estuarine and freshwater ecosystems: A 50-year perspective. Divers Distrib 26: 1780-1797.
- Bates, O. K. and Bertelsmeier, C. (2021). Climatic niche shifts in introduced species. Curr Biol 31: 1252-1266.
- Bates, O. K., Ollier, S. and Bertelsmeier, C. (2020). Smaller climatic niche shifts in invasive than non-invasive alien ant species. Nat Commun 11: 5213.
- Blackburn, T. M., Pyšek, P., Bacher, S., Carlton, J. T., Duncan, R. P., Jarošík, V. Wilson, J. R. U. and Richardson, D. M. (2011). A proposed unified framework for biological invasions. Trends Ecol Evol 26: 333-339.
- Blackburn, T. M., Bellard, C. and Ricciardi, A. (2019). Alien versus native species as drivers of recent extinctions. Front Ecol Environ 17: 203-207.
- Broennimann, O., Fitzpatrick, M. C., Pearman, P. B., Petitpierre, B., Pellissier, L., Yoccoz, N. G., Thuiller, W., Fortin, M. J., Randin, C., Zimmermann, N. E., Graham, C. H., Guisan, A. (2012). Measuring ecological niche overlap from occurrence and spatial environmental data. Global Ecol Biogeogr 21: 481-497.
- Cardador, L. and Blackburn, T. M. (2020). A global assessment of human influence on niche shifts and risk predictions of bird invasions. Global Ecol Biogeogr 29: 1956-1966.
- Chase, J. M. and Leibold, M. A. (2003). Ecological niche: linking classical and contemporary approaches. The University of Chicago Press, Chicago.
- Diagne, C., Leroy, B., Vaissière, A. C., Gozlan, R. E. and Courchamp, F. (2021). High and rising economic costs of biological invasions worldwide. Nature 592: 571-576.
- Fitzpatrick, M. C., Dunn, R. R. and Sanders, N. J. (2008). Data sets matter, but so do evolution and ecology. Global Ecol Biogeogr 17: 562-565.
- Fournier, A., Penone, C., Pennino, M. G. and Courchamp, F. (2019). Predicting future invaders and future invasions. P Natl Acad Sci USA 116: 7905-7910.
- Guisan, A., Petitpierre, B., Broennimann, O., Daehler, C. and Kueffer, C. (2014). Unifying niche shift studies: insights from biological invasions. Trends Ecol Evol 29: 260-269.
- Guisan, A., Petitpierre, B., Broennimann, O., Kueffer, C., Randin, C. and Daehler, C. (2012). Response to Comment on“Climatic Niche Shifts Are Rare Among Terrestrial Plant Invaders”. Science 338: 193.
- Guisan, A., Tingley, R., Baumgartner, J. B., Naujokaitis-Lewis, I., Sutcliffe, P. R., Tulloch, A. I. T., Regan, T. J., Brotons, L., Mcdonald-Madden, E. and Mantyka-Pringle, C. (2013). Predicting species distributions for conservation decisions. Ecol Lett 16: 1424-1435.
- Horn, H. S. (1966). Measurement of overlap in comparative ecological studies. Am Nat 100: 419-424.
- Kraus, F. (2009). Alien reptiles and amphibians: a scientific compendium and analysis. Springer, Dordrecht, The Netherlands.
- Li, Y. M., Liu, X., Li, X. P., Petitpierre, B. and Guisan, A. (2014). Residence time, expansion toward the equator in the invaded range and native range size matter to climatic niche shifts in non-native species. Global Ecol Biogeogr 23: 1094-1104.
- Liu, C. L., Wolter, C., Xian, W. W. and Jeschke, J. M. (2020). Most invasive species largely conserve their climatic niche. P Natl Acad Sci USA 117: 23643-23651.
- Liu, X., Blackburn, T. M., Song, T. J., Li, X., Huang, C. and Li, Y. M. (2019). Risks of Biological Invasion on the Belt and Road. Curr Biol 29: 499-505.
- Liu, X., Blackburn, T. M., Song, T. J., Wang, X. Y., Huang, C. and Li, Y. M. (2020). Animal invaders threaten protected areas worldwide. Nat Commun 11: 2892.
- Liu, X., Petitpierre, B., Broennimann, O., Li, X. P., Guisan, A. and Li, Y. M. (2017). Realized climatic niches are conserved along maximum temperatures among herpetofaunal invaders. J Biogeogr 44: 111-121.
- Losos, J. B. (2008). Phylogenetic niche conservatism, phylogenetic signal and the relationship between phylogenetic relatedness and ecological similarity among species. Ecol Lett 11: 995-1003.
- Olson, D., Dinerstein, M., E., Wikramanayake, E. D., Burgess, N. D., Powell, G. V. N., Underwood, E. C., D'Amico, J. A., Itoua, I., Strand, H. E., Morrison, J. C. et al. (2001). Terrestrial ecoregions of the worlds: A new map of life on Earth. Bioscience 51: 933-938.
- Parravicini, V., Azzurro, E., Kulbicki, M. and Belmaker, J. (2015). Niche shift can impair the ability to predict invasion risk in the marine realm: an illustration using Mediterranean fish invaders. Ecol Lett 18: 246-253.
- Peterson, A. T. (2011) Ecological niche conservatism: a time structured review of evidence. J Biogeogr 38: 817-827.
- Peterson, A. T. and Nakazawa, Y. (2008). Environmental data sets matter in ecological niche modelling: an example with Solenopsis invicta and Solenopsis richteri. Global Ecol Biogeogr 17: 135-144.
- Petitpierre, B., Kueffer, C., Broennimann, O., Randin, C., Daehler, C. and Guisan, A. (2012). Climatic Niche Shifts Are Rare Among Terrestrial Plant Invaders. Science 335: 1344-1348.
- Pianka, E. R. (1980). Guild structure in desert lizards. Oikos 35: 194-201.
- Pyšek, P., Hulme, P. E., Simberloff, D., Bacher, S., Blackburn, T. M., Carlton, J. M., Dawson, W., Essl, F., Foxcroft, L. C., Genovesi, P. et al. (2020). Scientists' warning on invasive alien species. Biol Rev 95: 1511-1534.
- Rödder, D. and Engler, J. O. (2011). Quantitative metrics of overlaps in grinnellian niches: advances and possible drawbacks. Global Ecol Biogeogr 20: 915-927.
- Rödder, D., and Lötters, S. (2009). Niche shift versus niche conservatism? Climatic characteristics of the native and invasive ranges of the Mediterranean house gecko (Hemidactylus turcicus). Global Ecol Biogeogr 18: 674-687.
- Schoener, T. W. (1970). Nonsynchronous spatial overlap of lizards in patchy habitats. Ecology 51: 408-418.
- Seebens, H., Blackburn, T. M., Dyer, E. E., Genovesi, P., Hulme, P. E., Jeschke, J. M., Pagad, H., Pyšek, P., Winter, M., Arianoutsou, M. et al. (2017). No saturation in the accumulation of alien species worldwide. Nat commun 8: 14435.
- Seebens, H., Essl, F., Dawson, W., Fuentes, N., Moser, D., Pergl, J., Pyšek, P., van Kleunen, M., Weber, E., Winter, M., and Blasius, B. (2015). Global trade will accelerate plant invasions in emerging economies under climate change. Global Change Biol 21: 4128-4140.
- Simberloff, D., Martin, J., Genovesi, P., Maris, V., Wardle, D., Aronson, J., Courchamp, F., Galil, B., García-Berthou, E. and Pascal, M. (2013). Impacts of biological invasions: what's what and the way forward. Trends Ecol Evol 28: 58-66.
- Tingley, R., Vallinoto, M., Sequeira, F. and Kearney, M. R. (2014). Realized niche shift during a global biological invasion. P Natl Acad Sci USA 111: 10233-10238.
- Wang, X. Y., Yi, T., Li, W. H., Xu, C. X., Wang, S. P., Wang, Y. P., Li, Y. M. and Liu, X. (2022). Anthropogenic habitat loss accelerates the range expansion of a global invader. Divers Distrib .
- Wiens, J. J., Ackerly, D. D., Allen, A. P., Anacker, B. L., Buckley, L. B., Cornell, H. V., Damschen, E. I., Davies, T. J., Grytnes, J. A., Harrison, S. P. et al. (2010). Niche conservatism as an emerging principle in ecology and conservation biology. Ecol Lett 13: 1310-1324.
引用格式:金龙, 张志新, 杜元宝, 廖文波, 栾晓峰, 刘宣. (2022). 外来入侵物种的气候生态位漂移及其量化方法.
Bio-101: e1010679. DOI:
10.21769/BioProtoc.1010679.
How to cite: Jing, L., Zhang, Z. X., Du, Y. B., Liao, W. B., Luan, X. F. and Liu, X. (2022). Quantification of Realized Climatic Niche Shift of Invasive Alien Species.
Bio-101: e1010679. DOI:
10.21769/BioProtoc.1010679.